DavidGraham
Veteran
The issue is mainly software, not hardware. A developer at Ubisoft once explained why games are hard to parallelize:Why hasn't that happened already? Multicore has been standard for two decades. If engines aren't scaling to the core-count effectively, there's a lot of potential sitting idle.
"there is usually a “hot path” of CPU updates - read the input, player + camera physics and animation - that need to happen every frame. Very hard to parallelize, without extra latency / memory. Single-core performance is still important"
"It’s about update order. To be able to say where the camera is, you have to run the animation of the player. To do that, you need to do the physics step. And so on.You can make these run in parallel, if you’re OK with latency - e.g. use the physics sim from the frame before"
"Mostly an observation from the last games I worked on. The bottleneck is a single-threaded chain of updates for the player, rather than the number of entities simulated / rendered. Animation + camera updates are surprisingly expensive, due to e.g. ray casts and others"
"As an example, a camera system can require hundreds of sphere casts to not feel janky. Predicting where it will move, smoothly avoiding obstacles, etc"
NVIDIA also published a blog targeting CPU thread optimizations in games, in short: more CPU threads are often actually detrimental to performance, NVIDIA advises the games to reduce the thread count of their worker pools to be less than the core count of modern CPUs:
Many CPU-bound games actually degrade in performance when the core count increases beyond a certain point, so the benefits of the extra threading parallelism are outweighed by the overhead
On high-end desktop systems with greater than eight physical cores for example, some titles can see performance gains of up to 15% by reducing the thread count of their worker pools to be less than the core count of the CPU
Instead, a game’s thread count should be tailored to fit the workload. Light CPU workloads should use fewer threads
Executing threads on both logical cores of a single physical core (hyperthreading or simultaneous multi-threading) can add latency as both threads must share the physical resource (caches, instruction pipelines, and so on). If a critical thread is sharing a physical core, then its performance may decrease. Targeting physical core counts instead of logical core counts can help to reduce this on larger core count systems
On systems with P/E cores, work is scheduled first to physical P cores, then E cores, and then hyperthreaded logical P cores. Using fewer threads than total physical cores enables background threads, such as OS threads, to execute on the E cores without disrupting critical threads running on P cores by executing on their sibling logical cores
for chiplet-based architectures that do not have a unified L3 cache. Threads executing on different chiplets can cause high cache thrashing.
Core parking has been seen to be sensitive to high thread counts, causing issues with short bursty threads failing to trigger the heuristic to unpark cores. Having longer running, fewer threads helps the core parking algorithms.
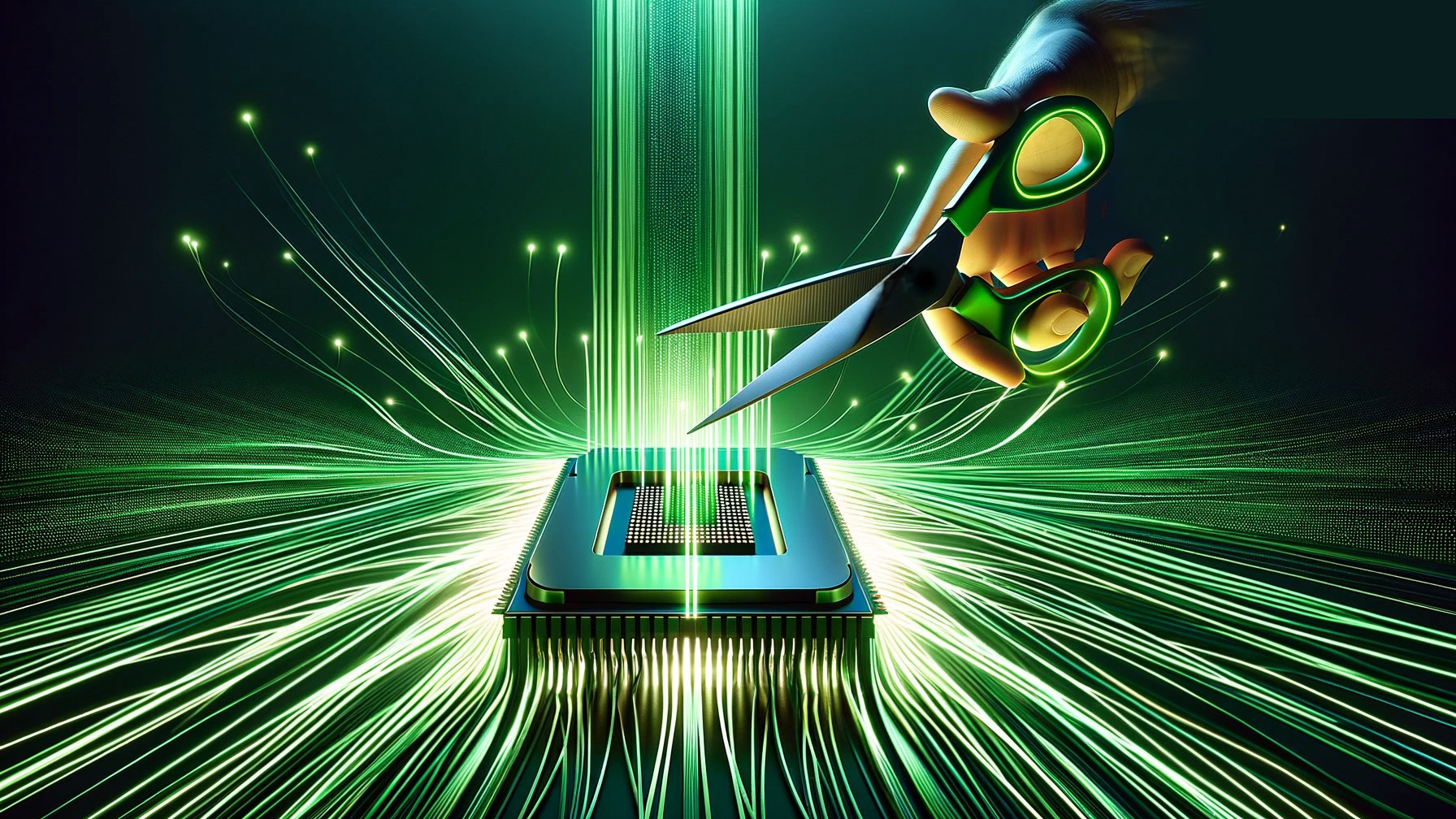
Limiting CPU Threads for Better Game Performance | NVIDIA Technical Blog
Many PC games are designed around an eight-core console with an assumption that their software threading system ‘just works’ on all PCs, especially regarding the number of threads in the worker thread…
Sebbi also talked about how engines and rendering paradigms often gets in the way of multi threading:
Let's talk about CPU scaling in games. On recent AMD CPUs, most games run better on single CCD 8 core model. 16 cores doesn't improve performance. Also Zen 5 was only 3% faster in games, while 17% faster in Linux server. Why? What can we do to make games scale?
History lesson: Xbox One / PS4 shipped with AMD Jaguar CPUs. There was two 4 core clusters with their own LLCs. Communication between these clusters was through main memory. You wanted to minimize data sharing between these clusters to minimize the memory overhead.
6 cores were available to games. 2 taken by OS in the second cluster. So game had 4+2 cores. Many games used the 4 core cluster to run your thread pool with work stealing job system. Second cluster cores did independent tasks such as audio mixing and background data streaming.
Workstation and server apps usually spawn independent process per core. There's no data sharing. This is why they scale very well to workloads that require more than 8 cores. More than one CCD. We have to design games similarly today. Code must adapt to CPU architectures.
On a two CCD system, you want to have two thread pools locked on these cores, and you want to push tasks to these thread pools in a way that minimizes the data sharing across the thread pools. This requires designing your data model and communication in a certain way.
Let's say you use a modern physics library like Jolt Physics. It uses a thread pool (or integrates to yours). You could create Jolt thread pool on the second CCD. All physics collisions, etc are done in threads which share a big LLC with each other.
Once per frame you get a list of changed objects from the physics engine. You copy transforms of changed physics engine objects to your core objects, which live in the first CCD. It's a tiny subset of all the physics data. The physics world itself will never be accessed by CCD0.
Same can be done for rendering. Rendering objects/components should be fully separated from the main game objects. This way you can start simulating the next frame while rendering tasks are still running. Important for avoiding bubbles in your CPU/GPU execution.
Many engines already separate rendering data structures fully from the main data structures. But they make a crucial mistake. They push render jobs in the same global job queue with other jobs, so they will all be distributed to all CCDs with no proper data separation.
Instead, the graphics tasks should be all scheduled to a thread pool that's core locked to a single CCD. If graphics is your heaviest CPU hog, then you could allocate physics and game logic tasks to the thread pool in the other CCD. Whatever suits your workload.
Rendering world data separation is implemented by many engines already. It practically means that you track which objects have been visually modified and bump allocate the changed data to a linear ring buffer which is read by the render update tasks when next frame render starts.
This kind of design where you fully separate your big systems has many advantages: It allows refactoring each of them separately, which makes refactoring much easier to do in big code bases in big companies. Each of these big systems also can have unique optimal data models.
In a two thread pool system, you could allocate independent background tasks such as audio mixing and background streaming to either thread pool to load balance between them. We could also do more fine grained splitting of systems, by investigating their data access patterns.